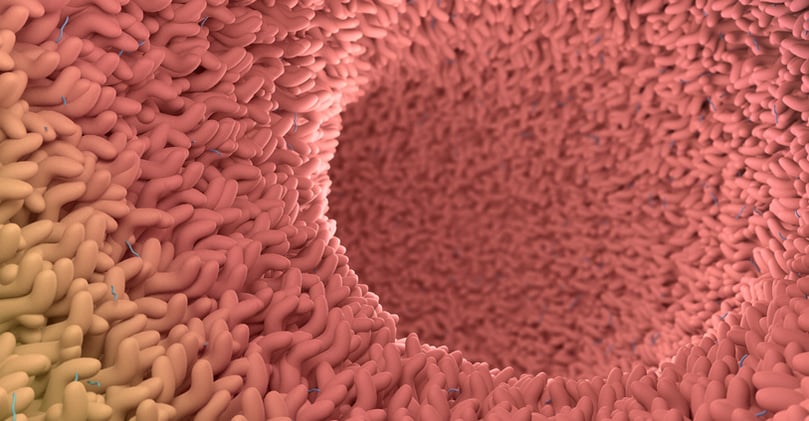
Gastrointestinal cancers are a heterogeneous group of cancers that can be caused by H. pylori bacterial or Epstein-Barr virus infection, chronic inflammation, or inherited or spontaneous genetic mutations. Advances in solid tumor immunotherapy for gastrointestinal cancers include the development of methods that improve the screening, evaluation, and development of more precise and robust treatments. Breakthroughs in basic and translational research are leading to better treatment options for gastrointestinal cancer, and CRISPR/Cas9-based (clustered regularly interspaced short palindromic repeats/CRISPR-associated protein 9) gene editing technology is at the forefront of these discoveries.
CRISPR/Cas9-based gene editing technology was originally identified in bacteria and archaea as an antiviral defense mechanism[1]. As a gene editing application, CRISPR/Cas9 uses the Cas9 nuclease to cleave specific sequences in the target DNA and introduces modifications such as knock-in or knock-out mutations[2]. The DNA sequence is targeted using a single-guide RNA (sgRNA) comprised of CRISPR RNA (crRNA) and trans-activating CRISPR RNA (tracRNA), and these RNAs can be expressed on the same plasmid as the Cas9 nuclease upon delivery into target cells. The crRNA/tracrRNA duplex can bind to the complementary sequence in the genomic DNA and recruit Cas9, which cleaves the target sequence and allows for DNA repair by either homology-directed repair or non-homologous end pairing. This repaired sequence is now a part of the genomic DNA and leaves virtually no trace of gene editing.
CRISPR/Cas9 gene editing has been used for several applications in oncology research and more recently to improve immune-oncology strategies. One of the earliest applications involves knockout screens in which a library of sgRNAs is used to transfect a pool of knockouts that can be used for gain-of-function or small molecule/drug screens. Genome-wide screens of cancer cell lines have been useful in identifying genes that are essential to cancer cell growth but can be prone to false-positive hits if the screens are not correctly designed or analyzed[3]. Genome-wide screens have also been used to identify genes linked to resistance, and such gene hits have been identified in a melanoma library screen when treating with the inhibitor vemurafenib[4]. Combinatorial CRISPR/Cas9 screens are now being used to target multiple genes, and this approach can be used for defining genetic or cellular pathways involved in cancer or characterizing drugs that target multiple genes[5].
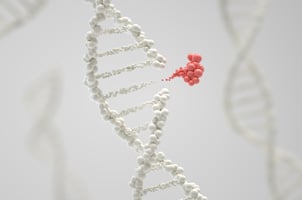
CRISPR/Cas9 has moved beyond in vitro cell culture and can be used for human organoid models for studying oncogenesis. ARID1A is one of the most frequently mutated genes in solid tumors, including gastric tumors[6]. ARID1A encodes a BAF complex subunit that normally targets BAF to AT-rich DNA sequences to regulate transcription. Mouse studies have suggested that ARID1A is involved in gastrointestinal cancer oncogenesis, but this was not validated in humans until a recent study that used CRISPR/Cas9-mediated depletion of ARID1A wild-type human gastric organoids. This ARID1A-knockout organoid system established a forward genetics model of gastric cancer that resembled gastric cancer phenotypically and identified BIRC5/Survivin as a key player in early tumorigenesis. This type of organoid model is not only useful for basic oncogenesis studies but can be used for small molecule inhibitor screens.
CRISPR/Cas9 screens can be used to validate mutations found in patients with gastrointestinal tumors. Activin A receptor type 2A (ACVR2A) is frequently mutated in patients with microsatellite instability-high gastric cancer, and a 2021 study used CRISPR/Cas9 to generate ACVR2A-knockout human gastric cancer cells[7]. These cells exhibited less aggressive proliferation, migration, and invasion in vitro, and patients with ACVR2A mutations had better prognoses than patients with wild-type ACVR2A. Gastrointestinal stromal tumors (GIST) have been typically characterized by activation mutations in the receptor tyrosine kinase KIT, but a 2022 study using genome-wide CRISPR screening identified the complementary chromatin-modifying complexes MOZ and Menin-MLL as being essential to GIST[8]. This finding highlights a trend in oncology research elucidating a role for chromatin regulation in tumorigenesis, which has the potential to be targeted therapeutically.
Beyond basic research, CRISPR/Cas9 is starting to be used in applications for clinical trials. A Phase II trial has recently launched that is using CRISPR/Cas9 to delete an intracellular checkpoint molecule called cytokine-induced SH2 (CISH) protein[9]. Preclinical studies have shown that CRISPR/Cas9-mediated deletion of CISH enhanced antitumor responses, and the Phase II trial is using patient-derived CRISPR-modified T cells to treat individuals with metastatic gastrointestinal tumors.
CAR T-cells (Chimeric Antigen Receptor) have been an incredible progress in personalized therapy targeting cancer.[10] However, different challenges hampered the availability, efficacy, and safety of this promising cell therapy. CRISPR-Cas9 technology can address many of these limitations. Desired genetic modification can be achieved with cellular precision engineering, leveraging CRISPR-Cas9-based gene editing. In addition, this approach can be exploited to overcome T-cell exhaustion, lack of CAR T-cell persistence, and toxicity related to cytokine release.
In particular, the implementation of CRISPR-Cas9 technology generated universal CAR T Cells resistant to PD1 Inhibition; or enhanced T cell function against bladder cancer disrupting CTLA-4.[11] In the oncolytic therapy field, it has been demonstrated that HSV-1-derived CD40L-armed oncolytic therapy by CRISPR-Cas9-based gene editing boosted endogenous immunity in pancreatic ductal adenocarcinoma.[12]
CRISPR/Cas9 gene editing will continue to transform basic and clinical research, immunotherapy, and cellular therapy providing effective and safe therapeutic strategies. Regulatory agencies are now developing frameworks to allow for the use of this revolutionary tool in a wide range of diseases.
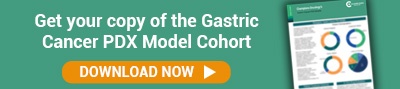
[1] Wiedenheft B, Sternberg SH, Doudna JA. RNA-guided genetic silencing systems in bacteria and archaea. Nature. 2012 Feb 15;482(7385):331-8.
[2] Barrangou R, Doudna JA. Applications of CRISPR technologies in research and beyond. Nat. Biotechnol. 2016;34(9):933-941.
[3] Munoz DM, Cassiani PJ, Li L, Billy E, Korn JM, Jones MD, Golji J, Ruddy DA, Yu K, McAllister G, DeWeck A. CRISPR screens provide a comprehensive assessment of cancer vulnerabilities but generate false-positive hits for highly amplified genomic regions. Cancer Discovery. 2016 Aug 1;6(8):900-13.
[4] Shalem O, Sanjana NE, Hartenian E, Shi X , Scott DA , et al. Genome-scale CRISPR-Cas9 knockout screening in human cells. Science. 2014. 343:84–87.
[5] Han K, Jeng EE, Hess GT, Morgens DW, Li A, Bassik MC. Synergistic drug combinations for cancer identified in a CRISPR screen for pairwise genetic interactions. Nat. Biotechnol. 2017 May;35(5):463-74.
[6] Lo YH, Kolahi KS, Du Y, Chang CY, Krokhotin A, Nair A, Sobba WD, Karlsson K, Jones SJ, Longacre TA, Mah AT, Tercan B, Sockell A, Xu H, Seoane JA, Chen J, Shmulevich I, Weissman JS, Curtis C, Califano A, Fu H, Crabtree GR, Kuo CJ. A CRISPR/Cas9-Engineered ARID1A-Deficient Human Gastric Cancer Organoid Model Reveals Essential and Nonessential Modes of Oncogenic Transformation. Cancer Discov. 2021 Jun;11(6):1562-1581.
[7] Yuza K, Nagahashi M, Ichikawa H, Hanyu T, Nakajima M, Shimada Y, Ishikawa T, Sakata J, Takeuchi S, Okuda S, Matsuda Y, Abe M, Sakimura K, Takabe K, Wakai T. Activin a Receptor Type 2A Mutation Affects the Tumor Biology of Microsatellite Instability-High Gastric Cancer. J. Gastrointest. Surg. 2021 Jan 8. doi: 10.1007/s11605-020-04889-9. Epub ahead of print.
[8] Hemming ML, Benson MR, Loycano MA, Anderson JA, Andersen JL, Taddei ML, Krivtsov AV, Aubrey BJ, Cutler JA, Hatton C, Sicinska E. MOZ and Menin-MLL Complexes are Complementary Regulators of Chromatin Association and Transcriptional Output in Gastrointestinal Stromal Tumor. Cancer Discovery. 2022 May 2.
[9] https://twin-cities.umn.edu/news-events/university-minnesota-opens-first-its-kind-clinical-trial-treat-metastatic-gi-cancers
[10] Jogalekar MP, Rajendran RL, Khan F, Dmello C, Gangadaran P, Ahn BC.Front Immunol. 2022 Jul 22;13:925985. doi: 10.3389/fimmu.2022.925985. eCollection 2022.
[11] Zhang W, Shi L, Zhao Z, Du P, Ye X, Li D, Cai Z, Han J, Cai J. Disruption of CTLA-4 expression on peripheral blood CD8 + T cell enhances anti-tumor efficacy in bladder cancer. Cancer Chemother Pharmacol. 2019;83:911–20.
[12] Wang R, Chen J, Wang W, Zhao Z, Wang H, Liu S, et al. CD40L-armed oncolytic herpes simplex virus suppresses pancreatic ductal adenocarcinoma by facilitating the tumor microenvironment favorable to cytotoxic T cell response in the syngeneic mouse model. J Immunother Cancer. 2022;10(1):e003809.