Cholangiocarcinoma is a type of cancer that originates from cholangiocytes, the cells that constitute the bile ducts in the liver, which carry bile from the liver to the small intestine. It is a rare and aggressive cancer that can be categorized into different subtypes based on histological and molecular characteristics.
Histologically, cholangiocarcinoma can be classified as intrahepatic, perihilar, or distal. Intrahepatic cholangiocarcinoma originates within the liver, while perihilar and distal cholangiocarcinoma develop in the ducts outside the liver. These subtypes have distinct clinical presentations and treatment approaches [1,2].
In this blog, discover the diverse nature of cholangiocarcinoma subtypes and learn about the specific clinical care required for each subtype.
Histological Characteristics and Clinical Care
Histological characteristics play a significant role in guiding clinical care for cholangiocarcinoma subtypes. Intrahepatic cholangiocarcinoma often presents as a solitary mass within the liver, while perihilar cholangiocarcinoma typically involves the bifurcation of the bile ducts. Distal cholangiocarcinoma commonly manifests as a tumor in the lower part of the bile duct near the small intestine.
The histological features of each subtype influence the choice of diagnostic tests, surgical interventions, and other treatment modalities. For example, surgical resection is often the primary treatment option for intrahepatic cholangiocarcinoma, while perihilar cholangiocarcinoma may require a combination of surgery and liver transplantation. Distal cholangiocarcinoma may be treated with surgery, radiation therapy, or systemic chemotherapy.
By understanding the histological characteristics of cholangiocarcinoma subtypes, healthcare professionals can provide appropriate clinical care to improve patient outcomes.
Cholangiocarcinoma Subtype-Specific Therapies
Each cholangiocarcinoma subtype requires specific therapies tailored to its unique characteristics. For intrahepatic cholangiocarcinoma, surgical resection is often the primary treatment approach, followed by adjuvant therapy such as chemotherapy or radiation therapy. Liver transplantation may be considered in selected cases.
Perihilar cholangiocarcinoma, on the other hand, often requires more complex surgical interventions, such as liver resection combined with bile duct resection and reconstruction. Liver transplantation may be an option for patients with advanced disease or underlying liver disease.
Distal cholangiocarcinoma may be treated with surgical resection, radiation therapy, or systemic chemotherapy. The choice of treatment depends on the extent of the tumor and the patient's overall health.
By tailoring therapies to the specific cholangiocarcinoma subtype, healthcare professionals can optimize treatment outcomes and improve patient survival rates.
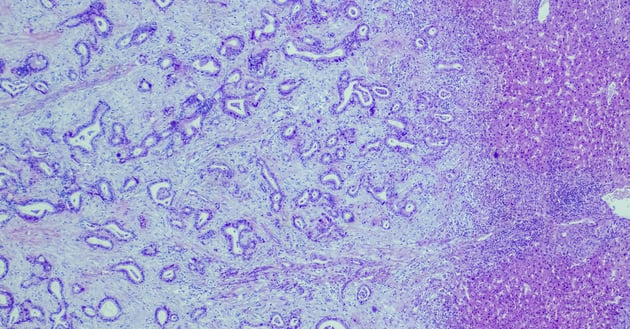
Molecular Profiling and Treatment Strategies
In addition to histological characteristics, molecular profiling has emerged as an essential tool for understanding cholangiocarcinoma subtypes and guiding treatment strategies. Molecular profiling involves analyzing the genetic and molecular alterations in cancer cells to identify potential targets for therapy.
Advancements in molecular profiling techniques have allowed researchers to identify specific biomarkers and genetic mutations associated with cholangiocarcinoma subtypes. This information helps in developing targeted therapies that can effectively inhibit the growth and spread of cancer cells.
Two targeted therapies are currently available for patients presenting tumors with FGFR2 fusions/rearrangements (infigratinib) or IDH1 (ivosidenib) mutation [3,4].
In general, treatment strategies for cholangiocarcinoma subtypes may include targeted therapies, immunotherapy, chemotherapy, and radiation therapy. Molecular profiling enables healthcare professionals to select the most appropriate treatment options based on the molecular characteristics of the tumor and the patient's overall health [5].
Future Directions in Cholangiocarcinoma Care
The field of cholangiocarcinoma care is rapidly evolving, with ongoing research and advancements in treatment options.
Efforts are being made to improve early detection methods for cholangiocarcinoma. Early diagnosis allows for timely intervention and increases the chances of successful treatment. Additionally, advancements in precision medicine and molecular profiling techniques hold promise for tailoring treatment plans based on the unique molecular characteristics of each patient's tumor.
In conclusion, the diverse nature of cholangiocarcinoma subtypes necessitates specific clinical care for optimal treatment outcomes. Through a comprehensive understanding of histological and molecular characteristics, healthcare professionals can provide personalized therapies and contribute to ongoing research efforts aimed at improving cholangiocarcinoma care.
