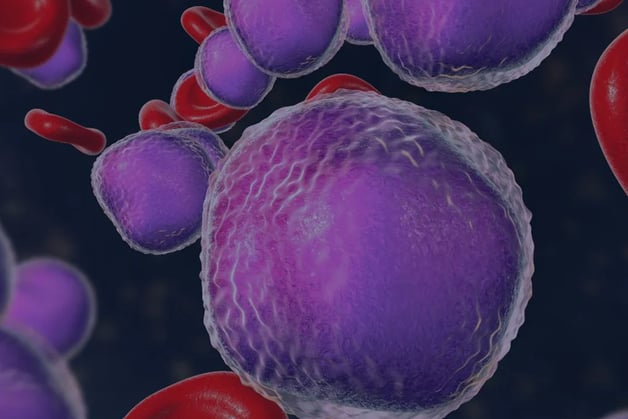
Did you know that Chronic Lymphocytic Leukemia (CLL) is a relentless adversary in the realm of hematologic malignancies? Characterized by the excessive accumulation of abnormal B lymphocytes, CLL presents a unique set of challenges for oncologists, hematologists, and cancer researchers. Despite advancements in targeted therapies and immunotherapies, achieving sustained remission remains elusive for many patients.
In this blog, we will explore the critical role preclinical CLL models play in the development of novel therapies. From understanding the disease's biology to facilitating the development of breakthrough treatments, these models are indispensable tools in our fight against CLL.
The Role of Preclinical CLL Models in Cancer Research
Preclinical CLL models are the unsung heroes of CLL research, providing a crucial bridge between laboratory discoveries and clinical applications. These models enable researchers to study the complex biology of CLL in a controlled environment, offering insights that are impossible to glean from human studies alone.
By replicating the disease in animals or in culture with preclinical CLL models, scientists can test the efficacy and safety of potential therapies before they reach clinical trials. This accelerates the drug development process and enhances our understanding of disease mechanisms. In essence, preclinical CLL models are the bedrock upon which modern CLL cancer therapies are built.
Overview of Current Preclinical CLL Models
The landscape of preclinical CLL models is diverse and continually evolving. Each model offers unique advantages and limitations, making choosing the right one for specific research objectives essential.
Cell line-derived Xenograft (CDX) Models
Xenograft models involve transplanting human CLL cells into immunodeficient mice. These preclinical CLL models allow for the study of human-specific disease characteristics and the evaluation of human-targeted therapies. However, the lack of a fully functional immune system in these mice limits the study of immune-based treatments.
Genetically Engineered Mouse Models (GEMMs)
GEMMs are designed to mimic the genetic aberrations found in human CLL. These preclinical CLL models provide a more accurate representation of the disease's progression and response to therapies. They are particularly valuable for studying the genetic and epigenetic factors driving CLL.
Patient-Derived Xenografts (PDXs)
PDXs involve implanting primary CLL cells from patients into mice and serially passaging to obtain stable in vivo models[1]. These preclinical CLL models retain genetic features of the original tumor, making them predictive of clinical outcomes, although the lack of a proficient immune system needs to be considered.
Using Preclinical CLL Models in Oncology Research
The latest therapies approved for CLL are a testament to the power of preclinical CLL models in developing revolutionary therapies. Pirtobrutinib (approved by the FDA at the end of 2023[2]) and lisocabtagene maraleucel (approved by the FDA for use in CLL in 2024[3]) were meticulously tested in preclinical CLL models before their clinical debut, ensuring their safety and efficacy in targeting CLL cells.
Pirtobrutinib is a highly selective, noncovalent Bruton tyrosine kinase inhibitor (BTKi). Preclinical testing of pirtobrutinib was conducted in several preclinical CLL models. CLL cell lines were used to assess target engagement, potency, cellular phosphorylation, and other cellular activity of the inhibitor[4, 5, 6]. Further studies in primary CLL cells and xenograft models confirmed pirtobrutinib's ability to kill CLL cells and reduce tumor burden[4, 6].
Lisocabtagene maraleucel is a CD19-targeted CAR-T cell therapy. Preclinical studies of lisocabtagene maraleucel involved in vitro and xenograft models to evaluate its ability to target and eliminate CLL cells[7, 8]. These preclinical CLL models provided crucial data on the therapy's potency, specificity, and potential side effects.
The success of these preclinical trials paved the way for pirtobrutinib and lisocabtagene maraleucel approval and use in clinical settings. These therapies are now transforming the treatment landscape for CLL and other hematologic malignancies.
The Impact and Future of Preclinical CLL Models in Developing Novel Therapies
The impact of preclinical CLL models on the development of new therapies cannot be overstated. They have accelerated the discovery of novel treatments, reduced the risk of adverse effects, and improved patient outcomes. However, the field is far from static.
Advancements in Model Precision
The future of preclinical CLL models lies in their ability to reproduce clinical characteristics and support personalized medicine. The use of primary patient-derived CLL models by direct injection of patients’ cells in mice without additional passages in the animals allows better preservation of tumor heterogeneity and patient population diversity[9], Patient-derived CLL models can be used in a preclinical trial format as well as for the testing of therapies on individual patient tumors, enabling tailored treatment strategies.
The development of such models in humanized mice, which possess a reconstituted human immune system, will further improve the robustness of these models as patients’ surrogates by enabling a deeper understanding of how therapies interact with the human immune system, leading to more effective treatments.
Integration of Computational Models
Integrating computational models with preclinical studies is another promising avenue. By simulating disease progression and treatment responses in silico, researchers can optimize experimental designs and predict outcomes more accurately. This synergy between computational and experimental approaches is poised to accelerate the development of next-generation CLL therapies.
The Importance of Continued Research and Innovation
Preclinical CLL models are the linchpin of CLL therapy development, providing invaluable insights and accelerating the transition from bench to bedside. As we continue to refine these models and integrate new technologies, the future of CLL treatment looks increasingly promising.
Champions Oncology's bank of preclinical CLL models includes primary models derived from pretreated and naive patients. With deep multi-omic and multimodal characterization and comprehensive clinical annotations, we strive to make our preclinical CLL models the best tool to accelerate your drug pipeline through reliable data and extensive expertise in the hematologic malignancies field. Contact us to speak with one of our experts.
